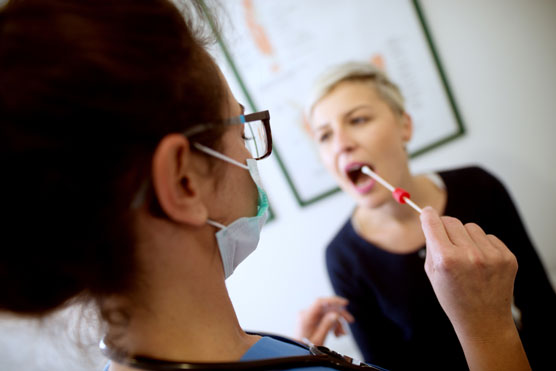
Direct-to-consumer genetic testing
Not too long ago, genetic testing was uncommon and was reserved for visits to a geneticist, genetic counselor, or other specialized healthcare provider. Now, a trip to the drug store, a little spit in a tube that you put in the mail, and that’s it. A few weeks or months later and you get to learn all about yourself! Of course, it’s not really that straightforward.Direct-to-consumer tests come in several varieties. The most common of these tests is genetic ancestry testing. Genetic ancestry testing involves testing individuals for sequence variations throughout the genome. The frequency of certain variants differs among different ancestral populations. Therefore, an analysis of the variants that a person carries and a comparison with previously tested individuals of known ancestry can give us an idea of where that person’s ancestors came from.
Popular direct-to-consumer genetic tests also include testing for health risks and common traits. The companies that offer these tests provide risk assessments for certain health conditions based on the particular sequence variants a person carries. For example, a specific variant in the gene for apoliprotein E (APOE) has been associated with an increased risk for developing late-onset Alzheimer disease (AD). Carrying the APOE e4 allele appears to increase one’s risk for developing AD late in life. Carrying two copies of the allele raises the risk even higher.
It is important to note that this is not a predictive test. It cannot tell you if you will develop AD or not, only whether you have an increased risk based on the versions of the APOE gene that you carry. Similar testing can tell you about your risk for conditions such as celiac disease, Parkinson’s disease, and diabetes, among others, but it cannot tell you whether or not you will get them.
At least one company offers testing for three variants in the breast cancer genes BRCA1 and BRCA2 (. The three variants that are a part of this test are found much more often in individuals of Ashkenazi (Eastern European) Jewish ancestry. Carriers of one of these three gene changes have a significantly increased risk for breast and ovarian cancer.
One of the things that is hard for many patients to understand about the BRCA1 and BRCA2 testing that is performed is that they will not be tested for the vast majority of mutations in these genes (thousands have been reported). So, if the person tested does not carry one of the three mutations included in the test, it does not rule out the possibility that they carry another mutation in one of these genes. And if the person is not of Ashkenazi Jewish ancestry, this portion of the test is of little value, since it is unlikely they would carry one of the three mutations anyway.
Whole exome sequencing
The genome is a complete set of chromosomes from an organism, including all coding and noncoding DNA. The exome contains all of the coding sequences found in the genome — all the exons in all of the genes. The exome, which is estimated to account for less than two percent of the genome, provides the instructions for creating the proteins in the body. Most mutations that cause genetic disorders are found in the exome.With advances in DNA sequencing technologies, it is now relatively straightforward (and not crazy expensive) to sequence the exomes of individuals in search of a genetic diagnosis. Consequently, whole exome sequencing has now entered mainstream genetics and is increasingly being used for patients who are suspected of having a genetic syndrome that has yet to be diagnosed. In many cases, patients have been on a long diagnostic odyssey with numerous medical evaluations, chromosome studies, and single gene tests, with no success. Whole exome sequencing allows for the testing of all known genes at the same time, regardless of the specific clinical features the person demonstrates.
Not only is whole exome sequencing providing diagnoses for those who were previously diagnosed with an “unknown genetic syndrome” (and many answers to the many questions of frustrated parents), it is providing a lot of information about the spectrum of features that can be associated with any particular disorder. We are finding out that the symptoms of genetic disorders can be quite varied. This means that someone may have a condition that would not have been suspected previously because they show features that are quite different than what is typically seen in individuals who have been diagnosed with the disorder.
One of the limitations of whole exome sequencing is the identification of incidental findings or genetic changes of unclear consequence. Incidental findings are those that are unrelated to why the testing was performed, such as identifying a gene changes that increases the risk for Alzheimer disease in a child who was tested because of intellectual disabilities. Genetic changes for which the effect is unclear typically are referred to as variants of unknown significance. It’s possible these changes could be the cause of some disorder, but it is also possible that they are completely harmless. The problem is there is not enough information about them yet to know which is true, and it is not always clear whether someone’s medical care should be altered based on the finding of a variant for which the effect is uncertain.
Whole exome sequencing is also being performed in large groups of individuals without genetic syndromes in order to determine how common different variants are in the population. The Exome Aggregation Consortium (ExAC) and the 100,000 Genomes Project are two collaborations that are collecting exome and/or genome data from a large number of individuals from a variety of ethnic backgrounds. Because of data like this, variants that were once classified as pathogenic (that is, as a disease-causing mutation) are now being reclassified as benign because we now know these variants are quite common in the general population (and, therefore, highly unlikely to cause any problems).
Whole genome sequencing
While whole exome sequencing involves determining the order of the nucleotides in all the exons in the genome, whole genome sequencing involves determining the sequence of the nucleotides in the entire genome. Whole genome sequencing was what was done during the Human Genome Project. And in the time since the Human Genome Project was completed, the ease with which sequencing can be performed and the significantly lowered cost have led to whole genome sequencing entering the clinic (just as with whole exome sequencing).The idea with whole genome sequencing is that it should theoretically be able to identify of the cause of rare genetic conditions that cannot be diagnosed using more traditional methods, or even whole exome sequencing. Scientists estimate that about 85 percent of disease-causing mutations involve the coding sequences of the genome. The remaining 15 percent or are expected to be located in noncoding sequences that are not covered in other types of tests. Whole genome sequencing could identify the previously unidentifiable mutations in those with undiagnosed genetic syndrome. Indeed, a project in the United Kingdom is allowing all children with serious illnesses to have whole genome testing. So far, the program has found that about 1 in 4 seriously ill kids had an underlying genetic syndrome. One case was a girl with a rare and severe form of epilepsy, and once her diagnosis was made, it was realized that the child had been taking medication that was known to aggravate that type of epilepsy. The mutation that caused this condition could have been found using more traditional approaches, but whole genome sequencing was able to provide a result in several weeks without having to undergo a diagnostic odyssey.
As with whole exome sequencing, two of the main concerns with whole genome sequencing are incidental findings and the identification of variants of unknown clinical significance.
Stem cell research
Stem cells may hold the key to curing brain and spinal cord injuries. They may be part of the cure for cancer. These little wonders may be the magic bullet to solving all sorts of medical problems, but they’re at the center of controversies so big that their potential remains unknown.You’ve probably guessed (or already knew) that one of the sources of stem cells for research is embryonic tissue — and therein lies the rub. However, in 2006, scientists figured out a way to turn multipotent stem cells (cells that can give rise to just a few, related cell types) from an adult organism into pluripotent stem cells (cells that can give rise to any of the cell types in the body of an organism). These stem cells are referred to as induced pluripotent stem cells. This discovery meant that it was now possible to collect stem cells from a patient, modify them, and return them to the patient, eliminating the chance of tissue rejection.
Currently, the most common use of stem cells is in hematopoietic stem cell therapy, which can be used to treat certain types of cancer and immune conditions. In hematopoietic stem cell therapy, the stem cells can be obtained from bone marrow, from the bloodstream, or from blood in the umbilical cord. This type of stem cell is a multipotent stem cell, which can give rise to any of the blood-related cell types (such as red blood cells and white blood cells).
Other uses of stem cells are being evaluated, with hopes that stem cell therapy will be able to help those with a number of different conditions, including neurodegenerative disorders and blindness. In fact, in 2018, researchers from England reported the results of a phase I clinical trial in which two patients with age-related macular degeneration were treated with stem cells. In age-related macular degeneration, specific light-sensitive cells in the retina die off, resulting in a progressive loss of central vision. In the trial, the eyes of both patients were injected with a patch of stem cells. Over the course of the following year, both patients showed dramatic improvement in their eyesight.
While stem cells in one form or another have yet to find their way into everyday medicine, ongoing studies are promising and it is likely just a matter of time.
When considering new or experimental medical therapies, it is important that the patient is aware of the history and experience of the provider and medical practice offering the treatment. Anyone considering medical treatment for a chronic condition should consult with a licensed and reputable healthcare provider.
The ENCODE Project
The goal of the Human Genome Project was to sequence the entire human genome. In 2001, a draft of the human genome sequence was published. And after about 13 years from start to finish (2 years ahead of schedule), the project was deemed complete. As a result of the project, we now know that there are approximately 22,000 genes (compared to the original prediction of 100,000 genes) and that coding sequences (the sequences that actually code for the protein products made by the genes) account for less than 2 percent of the entire human genome. We also have many new and improved tools for the analysis of genetic data. What we didn’t know is the function of the more than 98 percent of sequences — noncoding sequences that were once referred to as junk DNA.The Encyclopedia of DNA Elements (ENCODE) Project is a follow-up to the Human Genome Project. This project involves more than 30 research groups and more than 400 scientists from across the globe. The main goal of this project is to explore and determine the function of the noncoding sequences in the human genome. Research to date suggests that more than 80 percent of the noncoding sequences play a role in the regulation of gene expression.
Research also is attempting to determine whether differences in the expression of certain genes (as opposed to difference in gene sequence) can be linked with the development of specific genetic disorders. While most disease-causing mutations are found in the protein-coding portions of gene (the exons), some are found in non-coding regions of the genes (such as in the promoters or introns).
Scientists also expect that sequence variants located in other noncoding regions, including regions located distant to the gene itself (such as in enhancers or silencers), could affect gene expression and result in the development of genetic conditions.
Proteomics
Genomics is the study of whole genomes. Proteomics is the study of all the proteins an organism makes. Proteins do all the work in your body. They carry out all the functions that genes encode, so when a gene mutation occurs, the protein is what winds up being altered (or goes missing altogether). Given the link between genes and proteins, the study of proteins may end up telling researchers more about genes than the genes themselves!Proteins are three-dimensional. Proteins not only get folded into complex shapes but also get hooked up with other proteins and decorated with other elements such as metals. Scientist can’t just look at a protein and tell what its function is. However, if researchers can better understand each protein and what role it may play, proteins may be a big deal in the development of medicines and other treatments, because medications act upon the proteins in your system.
Cataloging all the proteins in your proteome isn’t easy, because researchers have to sample every tissue to find them all. Nonetheless, the rewards of discovering new drugs and treatments for previously untreatable diseases may make the effort worthwhile. Proteomics hasn’t made a big splash in clinical settings just yet — complexities and technological setbacks have slowed progress. However, like most things in genetics, it’s likely just a matter of time.
Gene chips
Technology is at the heart of modern genetics, and one of the most useful developments in genetic technology is the gene chip. Also known as microarrays, gene chips allow researchers to quickly determine which genes are at work (that is, being expressed) in a given cell.Gene expression depends on messenger RNA (mRNA), which is produced through transcription. The mRNAs get tidied up and sent out into the cell cytoplasm to be translated into proteins. The various mRNAs in each cell tell exactly which of the thousands of genes are at work at any given moment. In addition, the number of copies of each mRNA conveys an index of the strength of gene expression. The more copies of a particular mRNA, the stronger the action of the gene that produced it.
Gene chips are grids composed of bits of DNA that are complementary to the mRNAs the geneticist expects to find in a cell. It works like this: The bits of DNA are attached to a glass slide. All the mRNAs from a cell are passed over the gene chip, and the mRNAs bind to their DNA complements on the slide. Geneticists measure how many copies of a given mRNA attach themselves to any given spot on the slide to determine which genes are active and what their strength is.Gene chips are relatively inexpensive to make and can each test hundreds of different mRNAs, making them a valuable tool. One thing this screening can be used for is to compare mRNAs from normal cells to those from diseased cells (such as cancer). By comparing the genes that are turned on or off in the two cell types, geneticists can determine what’s gone wrong and how the disease may be treated. Scientists are also using microarrays to screen thousands of genes rapidly to identify specific mutations that cause disease, or to test patients for certain types of chromosome abnormalities.
Evolution of antibiotic resistance
Unfortunately, not all “next big things” are good. Antibiotics are used to fight diseases caused by bacteria. When penicillin (a common antibiotic) was developed, it was a wonder drug that saved many, many of lives. However, many antibiotics are nearly useless now because of the evolution of antibiotic resistance.Bacteria don’t have sex, but they still pass their genes around. They achieve this feat by passing around little circular bits of DNA called plasmids. Almost any species of bacteria can pass its plasmids on to any other species. Thus, when bacteria that are resistant to a particular antibiotic run into bacteria that aren’t resistant, the exchange of DNA endows the formerly susceptible bacteria with antibiotic resistance. Antibacterial soaps and the overprescribing of antibiotics make the situation worse by killing off all the nonresistant bacteria, leaving only the resistant kind behind. This can make illnesses that result from bacterial infections very difficult to treat. Consequently, scientists must continually work to develop new, more powerful antibiotics in an effort to stay one step ahead of the bacteria.
Circumventing Mother Nature
While you get your nuclear DNA (your autosomes and sex chromosomes) from both your mother and your father, your mitochondrial DNA (mtDNA) comes solely from your mother. So, conditions that are the result of changes in the mtDNA can only be inherited from mom. And if mom carries a mutation in her mtDNA, each of her children have a risk of inheriting it and developing a mitochondrial disorder, many of which are quite severe and potentially lethal. Well, a relatively new technique for preventing the inheritance of a mitochrondrial disorders is mitochondrial transfer (or mitochondrial replacement therapy).To minimize the chance of a child inheriting his mother’s mtDNA mutation and developing a mitochondrial disorder, scientists developed a procedure where the mother’s mitochondria could be replaced with the mitochondria from a donor, so the child would inherit someone else’s mitochondrial DNA but her mother’s nuclear DNA. In 2016, the birth of a child that resulted from this procedure was reported. The family had already had two children who had died from the mitochondrial disorder Leigh syndrome. The nucleus of one of the mother’s eggs (containing the nuclear DNA) was transferred into a donor egg that had the nucleus removed. The egg was then fertilized by sperm from the father and implanted in the mother. The couple had a healthy son who had very low levels of the mother’s mtDNA mutation and no clinical symptoms of Leigh syndrome.
Genetics from afar
For many, a referral to a geneticist or genetic counselor means traveling several hours (potentially to another state) and often having to navigate a large, unfamiliar city. It may also mean a very long wait, from the time of referral to the time of the actual appointment. In the entire state of Alaska, there is currently a single genetics clinic with a single clinical geneticist. And in some states, the demand far exceeds the availability; consequently, the wait for an appointment can be as long as 12 to 18 months. To improve access to genetics services, there has been a significant increase in the use of telegenetics.Telegenetics involves using either the telephone or videoconferencing to connect a patient with a genetics provider. In a virtual patient visit with a clinical geneticist, the patient attends a clinic at a local physician’s office. The visit requires a computer, high-speed internet, a good camera, a few specialized medical tools, and a provider onsite (such as a nurse or genetic counselor) to assist. The geneticist can perform a thorough evaluation while being hundreds of miles away. Even more common these days are telephone genetic counseling sessions. Genetic counselors can provide all aspects of genetic counseling (including pre-test and post-test counseling) while the patient enjoys the comforts of home.