Subsequently, the cell’s own DNA repair machinery can repair the break, inserting a new segment of DNA if a fragment of DNA with the desired sequence is provided.
Gene editing could be used in a couple of different ways. First, it could be used to replace a sequence with a disease-causing mutation with the normal sequence, thereby correcting the underlying cause of a patient’s condition. Another use could be to disrupt a gene that is being expressed and turn it off. This would be helpful for conditions in which there is an excess of expression that contributes to the disorder, such as oncogenes in cancer.
Technologies to perform gene editing have been around for more than 20 years; however, the different tools vary with respect to how well they work. Gene editing tools that have been developed include the following:
- Zinc finger nucleases (ZFNs): ZFNs use a specific type of DNA binding domain (referred to as a zinc finger domain) to recognize the target sequence. While they have been successfully used to modify various plants, they are not really used anymore because they frequently affected the wrong sequence.
- Transcription activator-like effector nucleases (TALENs): TALENs use a transcription activator-like effector DNA binding domain that can be customized to target a specific sequence. TALENs have a higher efficiency than ZFNs and have been used to alter the genomes of a variety of different organisms. It has also been used experimentally to correct mutations that cause human disease.
- CRISPR-Cas9 (pronounced “crisper cass 9”): The CRISPR-Cas9 system is the most recently introduced and appears to have the highest accuracy of any of the methods. The system uses a customizable RNA guide to target the sequence to be edited, along with a CRISPR-associated protein (Cas). Because of its efficiency and accuracy, this method is rapidly becoming the most widely used approach for performing gene editing.
The CRISPR-Cas9 gene editing system is also the method that has been hitting the headlines. The basics of this system are described in the following section. In addition, since the system could be used in either somatic cells (the cells of the body other than the eggs or sperm) or in the germline (the sex cells – eggs and sperm), the importance of this distinction is discussed. The promise that the CRISPR-Cas9 system holds for the future treatment of genetic diseases has also raised some significant concerns.
CRISPR-Cas9 gene editing
CRISPR stands for clustered regularly interspersed short palindromic repeats. Cas9 is the CRISPR-associated protein number 9. CRISPR-Cas9 is based on a defense system that occurs naturally in some bacteria.Scientists discovered that the DNA in these bacteria contained many short palindromic sequences. Palindromes are words or sequences that are the same both forward and backward, such as the word racecar or the DNA sequence CATAATAC.
In the bacteria, these short palindromic sequences flanked segments of DNA from a virus that had previously infected the cell. Basically, every time a bacterium was infected with a virus (and survived the infection), the bacterium saved a bit of the viral DNA by inserting it between the palindromic DNA sequences (see the following figure). It would then make a small piece of RNA from this sequence, which would then be attached to a Cas protein that is able to cut DNA into pieces. If the bacterium was infected again by the same virus, the armed Cas protein would recognize the viral DNA and destroy it immediately.
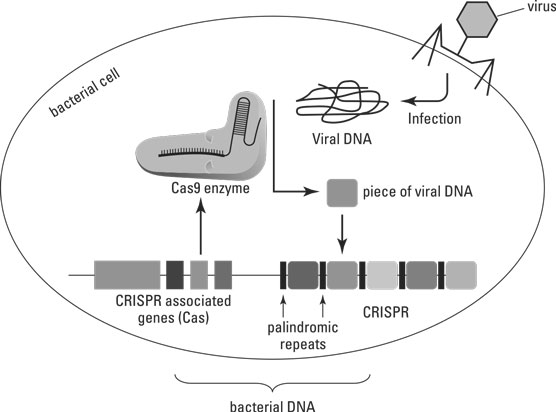
The CRISPR-Cas9 system for gene editing uses a short RNA guide sequence that can bind to a specific target sequence in the genome. This guide RNA also binds to the Cas9 protein. To use this system for correcting a gene known to carry a mutation, the Cas9 protein with the guide RNA attached could be introduced into a cell with the defective gene, along with fragments containing the normal gene sequence. Once the guide RNA-Cas9 complex binds to its target sequence in the genome, the Cas9 protein would cut the DNA at the target location.
The cells own DNA repair machinery could then be used to replace the defective copy of the gene with a correctly functioning version (without any mutation). Another potential use for this system would be to disrupt genes that are causing problems. For example, if a gene is producing a growth factor that is contributing to cancer cell division and proliferation, the guide sequence could target the gene and the attached Cas9 protein could cut it and stop it from making the growth factor.
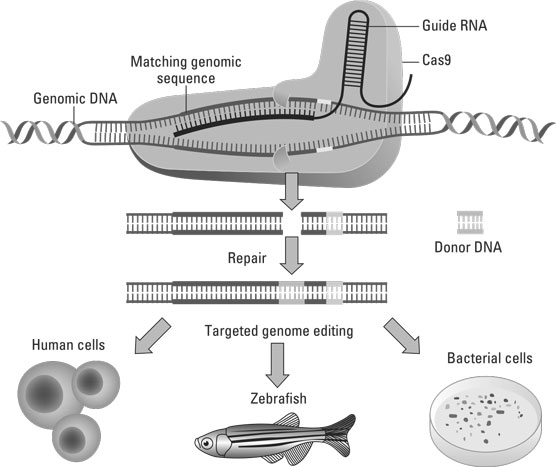
Germline versus somatic gene editing
Gene editing could be performed in a somatic cell (a body cell other than an egg or sperm), such that the edited sequence ends up only in the tissue of interest. In this case, the genetic change would not be passed on to subsequent generations. Alternatively, gene editing could be performed in a manner that results in the altered sequence being in all the cells of the body. In this case, the editing would need to occur in a germ cell (such as an egg or sperm) or in a one-cell zygote (fertilized egg). This would also lead to a genome alteration what would be passed onto future generations, something associated with a unique set of ethical issues.The ethics of gene editing
Both germline and somatic gene editing raise significant ethical concerns. In both cases, there are concerns about off-target editing (that is, when the wrong sequence gets changed, perhaps with devastating consequences). And like any new technology, there are definite concerns regarding the overall safety of such a procedure and the health implications for the organism in which the editing was performed (and potentially future offspring if germline editing is carried out).In addition, like all other genetic engineering technologies and some genetic testing approaches, there are worries regarding the potential to use these techniques to enhance one’s genetics, as opposed to treating a genetic condition.
Informed consent also triggers some very real apprehensions, since modifying someone’s germline involves performing the change before the person is born and before they can provide consent.
From a societal perspective, concerns have been raised regarding the equal access to such technologies and concerns related to how genetic diseases or traits are viewed. If therapies based on gene editing become clinically available, will everyone be able to afford them? Will this lead to a negative view of certain genetic disorders or traits, and an attempt to remove them from society?
Most of these concerns have been brought to the forefront since the first report demonstrating that genome editing in a human embryo is possible, which was published by a Chinese research group in 2015. This study, however, utilized an embryo that was not capable of developing to term. Later in 2015, there was a call for a voluntary worldwide moratorium on using CRISPR-Cas9 to alter the germline genome in humans. Despite this, a later report from China in 2018 described the use of gene editing to make twins who were immune to HIV infection. However, the genetic status of these individuals has not been verified.
At the time this article was written, a search on the clinicaltrials.gov website (which lists future, ongoing, and completed clinical trials) identified 19 different trials designed to study gene editing in somatic cells using CRISPR-Cas9. This included studies for the treatment of sickle cell disease, beta-thalassemia, and blood cell cancers (such as leukemia), and at least three of these trials have study sites in the United States. Little has been reported from these studies, but one researcher has stated that the first beta-thalassemia patient he treated hadn’t needed a blood transfusion in the four months since treatment was initiated. No trials were identified that involved modification of the human germline.